Nonlinear Nanophotonics
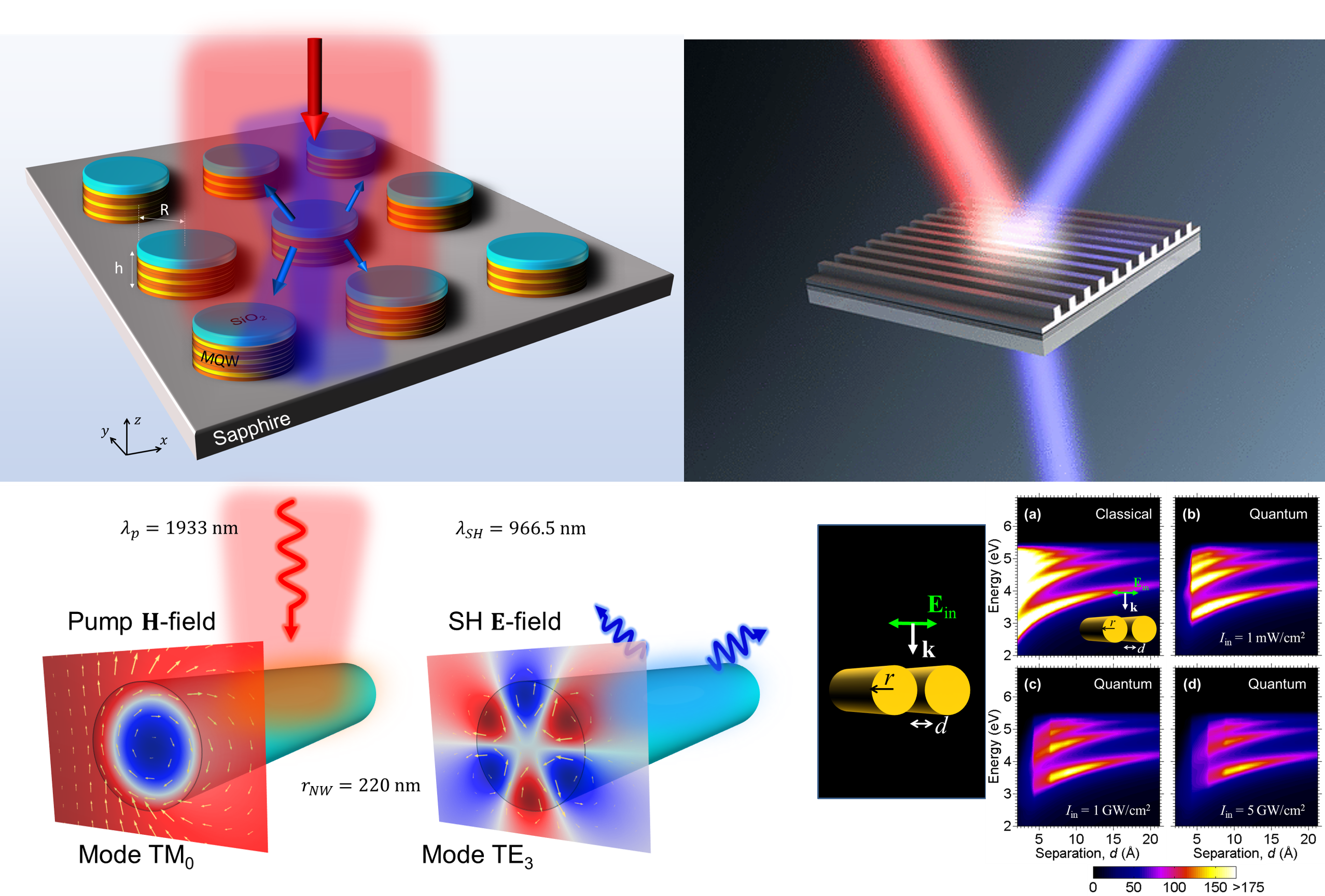
Over the years, progress in nanotechnologies and a steady miniaturization process have produced photonic and electronic devices with near-atomic size, raising questions about the applicability of classical electrodynamics. The classical approach adopts the following simplifications: (i) microscopic fields near individual atoms are averaged over a volume that contains myriad of atoms/dipoles, so that the medium loses its granularity and becomes a continuum necessitating the application of the macroscopic Maxwell’s equations; (ii) the ionic and electronic density at interfaces are step functions so that simple boundary conditions can be applied. This simplified picture is inadequate to describe systems with atomic-scale features or to predict electromagnetic effects associated with the presence of an electron cloud spilling out from surfaces. Moreover, the miniaturization trend has stimulated the growth of new research areas, such as nanoplasmonics, nonlinear plasmonics and quantum plasmonics.
A fundamental understanding of microscopic events occurring in the bulk and at interfaces of nanophotonic structures, and the effort to include these microscopic effects in accurate but numerically-efficient theoretical models, are crucial steps towards the advancement of these emerging fields. Important examples of these microscopic phenomena are nonlocal and quantum effects associated with light-excited conduction electrons. It has been proven that conduction-electrons nonlocality and quantum tunneling alter both linear and nonlinear optical response of nanostructures, for example by quenching and shifting plasmonic resonances in isolated and coupled metallic nanoparticles. Recently, the role of conduction electrons’ spill-out from metal surfaces has been studied with quantum hydrodynamic models and first-principle techniques that show good agreement with experiments. Moreover, generation of second- and third-harmonic light, as well as nonlinear absorption effects, originating from quantum tunneling in metal-insulator-metal systems, have been theoretically predicted via first-principle calculations and semiclassical theories.
This research activity is carried out to postulate and simulate new proof-of-principle devices that have novel transformational capabilities, using new designer/artificial materials, metamaterials, nanostructures, nanoantenna, photonic band gap structures, and to extend functionalities from the atomic level into the continuum, with the goal of enabling future, disruptive, opto-electro-plasmonic systems for sensors, energy-harvesting, and telecommunication devices.
Related Publications
- Sarma et al., Nano Letters 2021, 21, 1, 367–374
- Vincenti et al., Optics Express 2020, 28, 21, 31180-31196
- Rodriguez-Sune’ et al., Optics express 2021, in press
- Scalora et al, Physical Review A 2020, 101, 053828
- Carletti et al. Optics Express 2019, 27, 22, 32480-32489
- Sarma et al., ACS Photonics 2019, 6, 6, 1458–1465
- Scalora et al., Journal of the Optical Society of America B 2019, 36, 8, 2346-2351
- Hussain et al., Journal of Applied Physics 2019, 125, 105302
- de Ceglia et al., Applied Sciences 2019, 9, 16, 3381
Funding source US Army
Period 2019-2021